Optical dispersion is a fascinating phenomenon that occurs when light spreads out into its different colors or wavelengths. Different materials can bend light in distinct ways, leading to this dispersion effect.
The primary optical device known to produce optical dispersion is a prism, which separates light into its visible wavelengths, creating a spectrum.
Understanding how dispersion works is crucial in various applications, from creating beautiful rainbows in nature to improving the performance of optical systems. The interaction of light with different materials reveals much about the electromagnetic waves involved and how they behave when passing through various mediums.
This exploration not only captivates the imagination but also plays a vital role in fields such as telecommunications, where controlling light signals is essential for effective communication.
Basics of Optical Dispersion

Optical dispersion occurs when light separates into different colors as it passes through a medium. This phenomenon is crucial for understanding how various optical devices manipulate light.
Key concepts include the refractive index and the behavior of light as it interacts with materials.
Nature of Light and Dispersion
Light is made up of different wavelengths. When white light, which contains all colors, passes through a transparent medium like glass, it bends at different angles.
This bending occurs due to the refractive index, a measure of how much light slows down in a material. The angle of refraction varies for different wavelengths because they interact differently with the material.
Snell’s law describes this relationship mathematically. As a result, longer wavelengths (like red) bend less than shorter wavelengths (like blue). This difference leads to the separation of colors, creating effects such as rainbows when light refracts through rain droplets or a prism.
Measuring Dispersion
Dispersion can be quantified using measures such as the Abbe number, which indicates the degree of dispersion in a material. A low Abbe number suggests strong dispersion, meaning light separates more readily into its component colors.
Additionally, angular dispersion refers to the spread of light angles that occur based on wavelength differences. It’s influenced by factors like electric susceptibility, which affects how a material interacts with electric fields.
Understanding these measurements helps in designing optical systems where precise color separation is required, such as in cameras or projectors.
Understanding Dispersion in Media
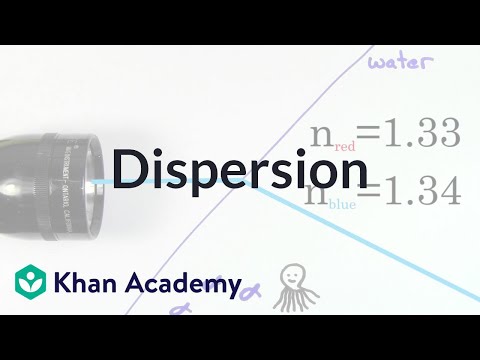
Dispersion in media occurs when different wavelengths of light travel at different speeds, leading to a separation of colors. This principle is important in various fields, including telecommunications and natural phenomena.
Optical Dispersion in Fibers
Optical fibers are key in telecommunications, allowing high-speed data transmission. In these fibers, chromatic dispersion refers to the spreading of light pulses due to varying speeds of different wavelengths.
This dispersion can be categorized into material dispersion and waveguide dispersion. Material dispersion occurs because different wavelengths interact differently with the fiber material, affecting their speed. Meanwhile, waveguide dispersion relates to the fiber’s structure, impacting how light travels through it.
Managing these types of dispersion is crucial for maintaining signal clarity. Techniques, such as using fibers with a low Abbe number, help reduce dispersion and enhance performance. By optimizing design, fibers can achieve minimal group-velocity dispersion, allowing for sharper signal transmission over long distances.
Dispersion in Natural Phenomena
Dispersion is often observed in nature, notably in the formation of rainbows. When sunlight enters raindrops, different wavelengths are refracted at varying angles due to their phase velocity differences. This causes white light to spread into its full spectrum of colors.
Another example is the dispersion of light in prisms, where electromagnetic radiation is separated into its constituent colors. Such phenomena illustrate how environmental factors contribute to optical effects. Understanding these patterns provides insight into light behavior and is vital in fields such as meteorology and optics.
Theoretical Aspects of Dispersion
Theoretical frameworks, such as the Kramers-Kronig relations, provide a basis for understanding the interaction between light and materials. These relationships describe how the real and imaginary parts of a material’s refractive index are connected, affecting light propagation.
Factors like material absorption and wavelength dependence also play a role in dispersion. For instance, longer wavelengths may experience different levels of absorption, influencing overall dispersion characteristics.
Additionally, modal dispersion happens in multimode fibers, where multiple light paths cause varying arrival times. This effect can lead to signal broadening, making it essential to consider during fiber optic design to ensure optimal signal quality and integrity.
Applications and Implications

Optical dispersion plays a significant role in various fields, influencing both technology and science. Understanding its applications helps reveal its importance in improving performance and functionality in optical devices. The following details explore key areas where dispersion is critical.
Dispersion in Optics and Photonics
Dispersion is essential in optics and photonics, affecting how light interacts with different materials.
For example, glass prisms are used in telescopes and microscopes to separate light into its component colors. This property enables the design of spectroradiometers, which measure light’s intensity across various wavelengths.
In laser systems, managing dispersion can enhance signal quality and reduce chromatic aberration. Conversely, unwanted dispersion can introduce issues like pulse broadening in optical fibers, leading to signal degradation over long distances. This phenomenon is critical when developing communication technologies, as it impacts data transmission efficiency.
Dispersion Management
Effective dispersion management is vital in optimizing optical systems, especially in high-speed communications.
It involves techniques to control and compensate for dispersion effects, improving performance in fiber optic networks.
Methods like positively chirped soliton waves help maintain signal integrity by counteracting the spread of light pulses.
Materials like flint glass can be designed with targeted dispersion properties to minimize aberrations in telescopes and other optical devices.
Understanding absorption and its impact on light behavior in the interstellar medium is also crucial.
Engineers and scientists develop advanced materials to mitigate dispersion effects, ensuring reliable operation in various applications, from consumer electronics to industrial systems.