Aberration in ray optics refers to the imperfection in an optical system that prevents it from producing clear images. This phenomenon occurs when light rays do not converge at a single point, resulting in blurred or distorted images that can significantly affect image quality.
Various types of aberrations, such as spherical and chromatic aberration, arise due to the design and materials used in lenses, altering how light is focused.
Understanding aberration is crucial for improving the performance of lenses in cameras, microscopes, and other optical devices. Engineers and scientists work diligently to minimize these optical aberrations, ensuring that images retain the clarity and detail desired in numerous applications.
By exploring the causes and effects of aberration, readers can gain insights into how optical systems function and the importance of precise lens design.
As the world of optics continues to advance, the study of aberration remains vital to enhancing image quality. This article delves into the different types of aberrations and their implications for optical systems, revealing the complex interplay between light and lens design that shapes our perception of the visual world.
Types of Aberrations

Aberrations in optics can be categorized into two main types: monochromatic and chromatic. Understanding these types is crucial for evaluating the performance of optical systems such as lenses.
Monochromatic Aberrations
Monochromatic aberrations occur when light of a single wavelength passes through a lens. These aberrations happen because of the lens geometry and how it refracts light.
Key types of monochromatic aberrations include:
-
Spherical Aberration: This occurs when light rays passing through the edges of the lens focus at different points than those passing through the center, causing a blurred image.
-
Coma: Coma leads to image distortion that appears comet-like, particularly evident when viewing off-axis points of light.
-
Astigmatism: Astigmatism results in different focal points for different planes, causing images to appear sharp in one direction and blurred in another.
-
Field Curvature: This aberration occurs when the image plane is curved instead of flat, leading to sharp focus at some points and blurriness at others.
-
Distortion: Distortion alters the shape of the image, making straight lines appear curved.
These aberrations can degrade the optical quality and clarity of images formed by lenses.
Chromatic Aberrations
Chromatic aberrations arise due to the dispersion of light, where different wavelengths are refracted by varying degrees. This results in color fringing around images. Chromatic aberration is split into two main types:
-
Axial Chromatic Aberration: This occurs along the optical axis and affects the focus of different colors. If red and blue light do not converge at the same point, it can produce a blurred image.
-
Lateral Chromatic Aberration: This affects colors at the edges of the lens. It causes different colors to appear offset from one another, particularly noticeable in high-contrast images.
In both cases, the refractive index of the lens material plays a significant role in the severity of chromatic aberration. Proper lens design can help minimize these effects and improve image fidelity.
Correcting Aberrations in Optical Systems

Aberrations in optical systems can significantly impact the quality of images produced by lenses, mirrors, and telescopes. Addressing these issues involves careful consideration of design parameters and the implementation of advanced correction techniques. This section explores the critical factors necessary for enhancing optical quality.
Design and Material Considerations
To minimize aberrations, lens design plays a vital role. Designers often use a combination of crown glass and flint glass in creating achromats. These materials help correct chromatic and spherical aberrations by allowing different wavelengths of light to converge more accurately.
The configuration of the entrance pupil and exit pupil is also important. By adjusting these parameters, designers can optimize the path of light through the system.
Additionally, the use of computer-aided design software facilitates advanced modeling, enabling effective identification and minimization of wavefront errors in the system.
Using precise spot diagrams derived from ray tracing helps visualize the effect of lens shapes and positions on light paths, aiding in better design. Each of these elements contributes significantly to imaging quality.
Techniques and Technologies
Several advanced techniques are used to correct aberrations in optical systems. Adaptive optics is one prominent technology. It adjusts the optical elements in real-time to compensate for distortions caused by atmospheric turbulence or imperfections in the optics.
Furthermore, specialized coatings can be applied to lens surfaces. These coatings reduce reflections and improve light transmission, enhancing the overall performance.
The integration of digital signal processing with optical systems provides real-time feedback, allowing for dynamic adjustments to improve image quality.
Innovative lens shapes, such as aspherical designs, also help reduce various types of aberrations. By deviating from standard spherical shapes, these lenses can focus light more precisely, resulting in sharper images. Each approach offers specific advantages in achieving high optical performance.
Practical Applications and Considerations
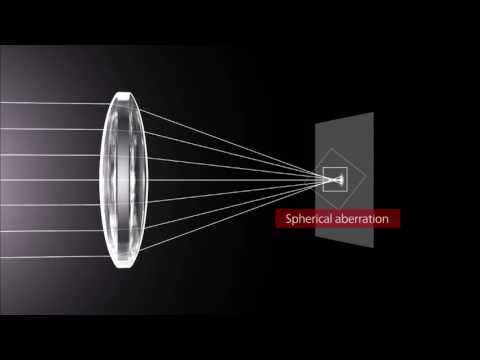
Aberrations affect various optical devices, including camera lenses, microscopes, and telescopes. Understanding these distortions helps in designing systems that produce clearer images.
In camera lenses, aberrations can lead to blurriness or color fringing. Manufacturers often use special glass and design techniques to minimize these effects. This is essential for achieving high-quality photographs.
Microscopes depend on precise imaging for biological research. Aberrations can obscure fine details, impacting analyses. Using corrected optics can improve clarity and allow for better observation of specimens. More information about microscopes can be found in related articles.
In telescopes, aberrations can affect astronomical observations. They can cause stars to appear blurry or distorted. By optimizing the optical path length, astronomers can enhance the focus and improve image quality. For more details, explore articles on telescopes.
Considerations for optical systems include the image space and object space. Designers must balance the two to reduce aberrations while maintaining usability. For example, achieving a sharp focus on distant stars in telescopes requires careful alignment of optics.
Theoretical Foundations and Complex Effects

Understanding optical aberrations requires exploring wavefront theory and the limitations of classical optics. These concepts shed light on how light behaves as it travels and interacts with various optical systems.
Wavefront Theory and Diffraction
Wavefront theory examines how waves propagate through space. Light can be analyzed as a series of wavefronts. An ideal wavefront travels in a straight line from a point source.
When these wavefronts encounter obstacles or apertures, diffraction occurs, causing the light to bend and produce interference patterns.
The diffraction limit defines the finest detail that can be resolved by an optical system, often represented by the Airy disk. This disk demonstrates that even perfect optical systems cannot eliminate all aberrations.
Notable types of wavefront aberrations include defocus, which occurs when light rays from an object converge incorrectly at the focal point. Ray aberrations, like longitudinal and transverse ray aberration, describe the deviation of rays from their ideal paths. This results from variations in the curvature of wavefronts across the optical axis, meaning that not all rays focus at the same point.
Beyond Paraxial Optics
Paraxial optics is limited to small angles and shallow deviations from the optical axis. Here, ray approximations hold true, but real systems often operate beyond these ideal conditions.
As angles increase, skew rays and other complex interactions emerge, leading to more significant aberrations.
In this non-paraxial regime, understanding the geometry of light paths becomes vital. The increasing deviation means traditional geometric optics approximations fail.
Consequently, considering the interplay of rays at wider angles becomes essential for precise modeling.
When delving deeper into phenomena like wave interference and diffraction focus, optical designers gain insight into the performance of systems. This awareness allows for the adjustment and correction of optical components, ultimately enhancing image quality and system effectiveness.