Dispersion in optics is a fascinating phenomenon that refers to how light spreads out into different colors based on its wavelength.
There are several types of dispersion, including material dispersion, waveguide dispersion, and intermodal dispersion, each influencing how light behaves in distinct ways.
Understanding these types helps explain why we see beautiful rainbows and how various optical devices, like prisms, operate to separate light into its color spectrum.
In material dispersion, the refractive index of a medium changes with wavelength, causing different colors of light to bend at different angles. This is why a prism can break white light into a spectrum of colors.
Similarly, waveguide dispersion occurs in optical fibers, where the design leads to different light wavelengths traveling at varying speeds, impacting communication signals.
Another critical type is intermodal dispersion, which happens in multimode optical fibers. Here, light rays take different paths through the fiber, resulting in signal spreading over distances.
The Nature of Light and Dispersion

Light is an essential aspect of the universe, and its behavior is key to understanding various optical phenomena. One significant process involving light is dispersion, which occurs when different wavelengths, or colors, of light spread apart.
This section covers the electromagnetic spectrum of light and the fundamental principles of light dispersion.
The Electromagnetic Spectrum of Light
Light is part of the electromagnetic spectrum, which includes a range of wavelengths and frequencies. The spectrum is divided into several categories: radio waves, microwaves, infrared, visible light, ultraviolet, X-rays, and gamma rays.
Visible light is the small portion of the spectrum detectable by the human eye. Its range varies from about 400 nm (violet) to 700 nm (red). Each color in this spectrum corresponds to a specific wavelength:
- Violet: ~400 nm
- Blue: ~450 nm
- Green: ~520 nm
- Yellow: ~580 nm
- Red: ~700 nm
This spectrum is essential for understanding how different wavelengths behave when interacting with materials, which leads to phenomena such as dispersion.
Basics of Light Dispersion
Dispersion occurs when light passes through a medium, causing it to separate into its constituent colors. This happens because each wavelength travels at different speeds in the medium.
For example, in glass, blue light (shorter wavelength) refracts more than red light (longer wavelength). This differential bending creates a spectrum of colors, commonly seen in rainbows and prisms.
The speed of light also varies with the medium; it travels faster in air than in water or glass. When white light enters a prism, it splits into a range of colors, demonstrating the process of light dispersion clearly.
Mechanisms of Optical Dispersion
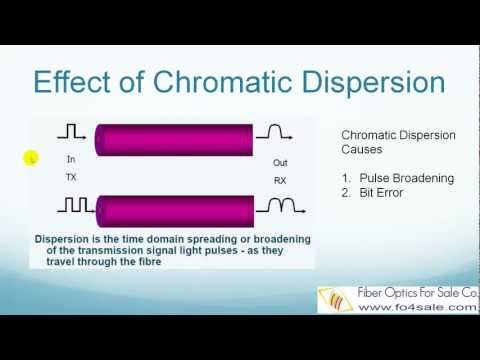
Optical dispersion occurs when light waves separate into different colors as they pass through various materials. Two primary mechanisms influence this process: material dispersion and modal dispersion, particularly within optical fibers. Understanding these mechanisms helps clarify how light behaves in different environments.
Material Dispersion and Refractive Index
Material dispersion arises from variations in the refractive index of a medium. The refractive index determines how much light bends as it enters a material. Different wavelengths of light travel at different speeds within the same medium, causing separation, or dispersion.
In optical applications, such as using prisms, this effect creates a spectrum of colors when white light passes through. The spectrum forms because shorter wavelengths (blue light) refract more than longer wavelengths (red light).
This principle is crucial for understanding light behavior in technologies like microscopes and optical fiber communication systems, where precise control over light dispersion enhances performance.
Modal and Waveguide Dispersion in Optical Fibers
In optical fibers, modal dispersion and waveguide dispersion are significant. Modal dispersion occurs in multimode fibers. Here, light pulses travel through various modes, or paths, leading to differences in arrival times at the end of the fiber. This results in signal spreading.
Waveguide dispersion relates to how light behaves within the fiber’s structure, including the core and cladding. In single-mode fibers, waveguide effects are more pronounced, allowing less dispersion and supporting higher data rates.
On the other hand, chromatic dispersion can also affect signal integrity in both types of fibers. Understanding these types of dispersion is essential for designing effective fiber optics systems.
Types of Dispersion in Optical Systems
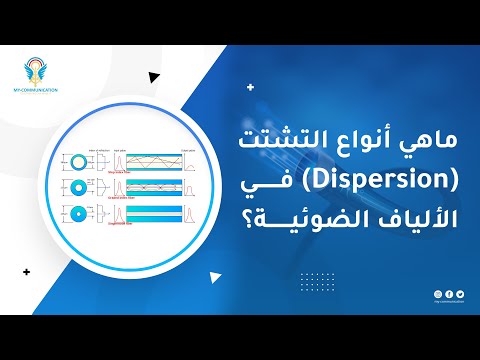
Dispersion in optical systems refers to how different wavelengths of light can spread apart when passing through various materials. This phenomenon affects many applications, particularly in devices like prisms and optical fibers. Understanding specific types of dispersion helps in effectively using these systems.
Chromatic Dispersion in Prism and Spectrometers
Chromatic dispersion occurs when light separates into its component colors as it passes through a prism. This happens due to varying angles of refraction for different wavelengths. For example, blue light bends more sharply than red light, creating a spectrum, which can even produce a rainbow effect.
Spectrometers utilize this principle to analyze light. They measure different wavelengths and their intensities. The Abbe number of a material indicates how much it will disperse light. A lower Abbe number means higher dispersion, which is crucial for accurate measurements in scientific applications.
Proper control of chromatic dispersion is essential for data transmission in optical fibers, ensuring that signals maintain their clarity over distances.
Polarization Mode Dispersion in Communications
Polarization mode dispersion (PMD) occurs in optical fibers and affects data transmission. Different light rays can have distinct polarization states, which leads to varying propagation speeds. This results in signal spreading over time, creating distortion.
In communications, PMD can diminish data rates and signal quality.
Engineers strive to minimize PMD by optimizing fiber design and utilizing specific polarization-maintaining fibers. Understanding these impacts allows for improved communication systems. By addressing PMD, networks can achieve higher data rates and enhanced reliability, making them critical for modern optical communications, including high-speed internet.
Dispersion Effects and Applications

Dispersion in optics plays a crucial role in various technologies and everyday applications. Understanding how different wavelengths of light behave when they travel through different mediums helps in enhancing communication systems and creating innovative optical devices.
Dispersion in Optical Communication Systems
In optical communication systems, dispersion can impact the quality of signals transmitted through optical fibers. When light travels through these fibers, different wavelengths can travel at different speeds, leading to signal spreading. This phenomenon is known as chromatic dispersion.
Two types of dispersion affect optical systems: material dispersion and waveguide dispersion. Material dispersion occurs due to the variation of the refractive index with wavelength in the fiber material. Waveguide dispersion is caused by how light is confined within the fiber core.
When signals spread, it can lead to overlap and distortion, which affects data integrity. To combat this, techniques such as dispersion compensation are employed. This includes adjusting the design of optical fibers and using specialized devices to maintain signal clarity over long distances.
Practical Uses of Dispersion Effects
Dispersion is not just a challenge; it also has numerous practical applications.
In everyday life, the dispersion of light creates beautiful phenomena such as rainbows. This effect occurs due to the separation of white light into its constituent colors when passing through water droplets.
In technology, dispersion is utilized in creating spectrometers that analyze the properties of materials by separating light into its wavelengths. These devices are crucial in fields such as chemistry and astronomy.
Moreover, understanding the effects of dispersion enables better designs in fiber optics, improving the performance of data communication networks.
It helps in tuning the electric susceptibility of materials, allowing scientists to enhance signal transmission through the interstellar medium and beyond.