Radio frequency, often abbreviated as RF, plays a crucial role in various scientific fields, especially in telecommunications and environmental studies. RF stands for both radio frequency and retention factor, depending on the context.
In radio engineering, it refers to the electromagnetic waves used for communication, while in chemistry, it signifies the ratio of the distance traveled by a substance in chromatography.
Understanding RF’s applications can lead to exciting insights. For instance, radio frequency is essential for technologies like mobile phones, Wi-Fi, and radar systems. On the other hand, the retention factor provides important data in laboratory settings, helping scientists analyze mixtures and understand their properties.
As RF continues to evolve with advancements in technology, exploring its different meanings becomes increasingly relevant. This article will delve deeper into the various interpretations of RF in science, making it easier for readers to grasp its significance in both communication and research fields.
RF in Chromatography
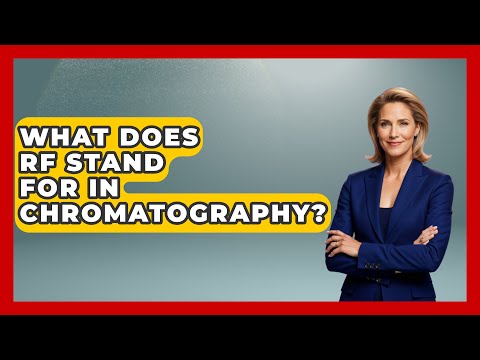
In chromatography, RF values play a crucial role in analyzing the movement of substances.
Understanding the principles, calculations, and interpretations of RF values helps identify and separate various compounds effectively.
Principles of RF in Chromatography
The RF value, known as the retardation factor, indicates how far a component travels in a chromatographic system compared to the solvent front. It is defined as:
RF = Distance traveled by the analyte / Distance traveled by the solvent front
In this context:
- Stationary Phase: The material that remains fixed, such as silica gel or paper.
- Mobile Phase: The solvent that moves through the stationary phase, carrying the analytes with it.
The polarity of both the mobile phase and the analyte affects how quickly each component moves. Polar compounds tend to interact more with polar stationary phases, resulting in lower RF values.
Conversely, non-polar or hydrophobic compounds may exhibit higher RF values as they migrate further with the non-polar solvent.
Calculating RF Values
To calculate the RF value, one must measure the distances traveled by the analyte and the solvent front from a starting point, usually the origin.
For instance, if the solvent front moves 10 cm and the analyte moves 7 cm, the RF calculation would be:
RF = 7 cm / 10 cm = 0.7
A high RF value (closer to 1) means the substance moved nearly as far as the solvent front. This indicates a high solubility in the mobile phase.
In contrast, a low RF value suggests the analyte has a strong interaction with the stationary phase. This method is widely used in techniques like Thin Layer Chromatography (TLC) and paper chromatography.
Interpretation of Chromatograms
Chromatograms visually represent the separation of components in a sample. Each spot on the chromatogram corresponds to a different analyte, with its position indicating its RF value.
For example, if the RF value for Sample 1 is calculated to be 0.5, that means it moved half the distance of the solvent front.
To interpret the results, one can compare the RF values of different analytes. Matching RF values can indicate similar chemical properties.
Factors such as temperature and solvent choice can also influence these values. By analyzing these characteristics, scientists can gain insights into the mixture’s composition and make informed decisions about further experimentation.
RF in Physics and Engineering
Radio Frequency (RF) plays a crucial role in various scientific fields, particularly in physics and engineering. It encompasses a range of frequencies used for different applications, including communication and power transfer. Understanding RF involves exploring its basics, applications, and safety guidelines related to exposure.
Basics of Radio Frequency
Radio Frequency refers to the oscillation of electromagnetic radiation within a specific frequency range, typically from about 20 kHz to 300 GHz. This range is significant for different forms of communication and transmission.
RF energy travels as waves and can propagate through various media. Devices like antennas and waveguides are designed to harness these frequencies effectively. Basic concepts involve understanding wavelength, frequency, and the behavior of electromagnetic fields.
RF is essential in technologies such as radio, television, and mobile communications.
RF Application in Technology
RF technology is foundational for modern communication systems. It is utilized in wireless communications, broadcasting, radar, and even remote sensing. Devices like cell phones, GPS, and Wi-Fi rely heavily on RF signals.
To illustrate, mobile phones operate around 900 MHz to 2.5 GHz, enabling many users to communicate simultaneously. Radar systems employ RF for detecting objects, crucial in aviation and weather forecasting. The following are notable RF applications:
- Wireless Communication: Uses RF signals to transmit data between devices without direct connections.
- Broadcasting: Transmits audio and visual content to a wide audience.
- Remote Sensing: Uses RF waves to gather data from distant locations, such as in satellite imaging.
Safety and RF Exposure
Safety regarding RF exposure is a significant topic of concern. RF energy, like any form of electromagnetic radiation, can pose health risks with prolonged exposure.
Research indicates that high levels of RF exposure can lead to adverse effects, including thermal and non-thermal impacts on human health. For this reason, regulatory bodies establish guidelines to limit exposure.
It is essential to use devices within recommended limits and ensure proper shielding in RF environments, especially in industrial settings. Simple precautions include keeping a safe distance from high-power antennas and using appropriate protective gear when necessary. Awareness and adherence to safety standards can greatly mitigate potential risks associated with RF exposure.
RF in Medicine and Biology
RF plays a critical role in both medicine and biology, particularly in diagnosing diseases and understanding biological processes. This section discusses the importance of Rheumatoid Factors, their applications in diagnostics, and how RF is utilized in biotechnology.
Understanding Rheumatoid Factors
Rheumatoid Factors (RF) are autoantibodies that the immune system produces against the body’s own tissues. They are commonly associated with rheumatoid arthritis (RA) and can be present in the blood of individuals with this condition.
In addition to RA, RF can also indicate other autoimmune diseases and even some infections. The presence of RF is important for diagnosing rheumatic fever and other related conditions. Understanding RF helps clinicians assess disease activity and determine appropriate treatment plans.
Applications of RF in Diagnostics
RF testing is a valuable tool in diagnosing rheumatoid arthritis and other autoimmune disorders. A positive RF test can enhance the certainty of an RA diagnosis, though it is not exclusive to this disease.
Doctors often use RF tests alongside other markers, such as anti-citrullinated protein antibodies (ACPAs), to get a comprehensive view of a patient’s condition. Negative RF results can also provide insight, suggesting that further evaluation is needed.
Common diagnostic methods include blood tests and clinical evaluations to assess the level of RF. This helps in monitoring disease progression and treatment response.
RF Utilization in Biotechnology
In biotechnology, RF is important for research and development of treatments targeting autoimmune diseases. Scientists study RF to understand its role in immune response and inflammation.
Research has focused on manipulating RF for potential therapeutic applications. By targeting RF, new medications and biologics can be developed to better manage conditions like rheumatoid arthritis.
Additionally, RF can be used in bioassays to monitor immune reactions in research settings. This helps in developing vaccines and therapies to boost immune responses against various diseases.
Through these applications, RF is significant not only in clinical settings but also in advancing medical research and biotechnological innovations.
Chemical and Isotopic Significance of RF
The term RF holds importance in both the realm of chemistry and in analytical techniques like mass spectrometry. Understanding these aspects helps clarify its various applications and significance in scientific study.
Rutherfordium (Element with Symbol RF)
Rutherfordium is a synthetic element with the symbol RF and atomic number 104. It was first discovered in the 1960s by teams in both the United States and the Soviet Union. Named after the physicist Ernest Rutherford, it is part of the actinide series in the periodic table.
This element is highly radioactive and has limited availability, making it difficult to study extensively. Its most stable isotope, Rutherfordium-267, has a half-life of about 1.5 hours. The chemical properties of Rutherfordium are not fully understood due to its short lifespan.
However, it is predicted to behave similarly to other group 4 elements, such as zirconium and hafnium, with potential uses in advanced materials and nuclear research.
RF Analysis in Mass Spectrometry
In mass spectrometry, RF can refer to the radio frequency energy used to manipulate ions within the mass analyzer.
The RF field helps in stabilizing the ions while they are being analyzed. This technique is crucial for identifying the mass-to-charge ratio of ions, allowing scientists to determine the molecular structure of compounds.
The RF field can also assist in trapping ions to improve detection sensitivity.
For example, in quadrupole mass spectrometers, varying RF voltages create an electric field that selectively stabilizes certain ions. This separation enables precise quantification of complex mixtures, enhancing the effectiveness of chemical analysis and research.